How to Buy Microscopy Equipment & Reagents
6 Dec 2024
This essential microscopy guide provides you with all the information you need to make the right decisions when investing in a microscope. Learn about key factors and application considerations for your imaging needs. Plus, read reviews from your peers to help you buy with confidence.
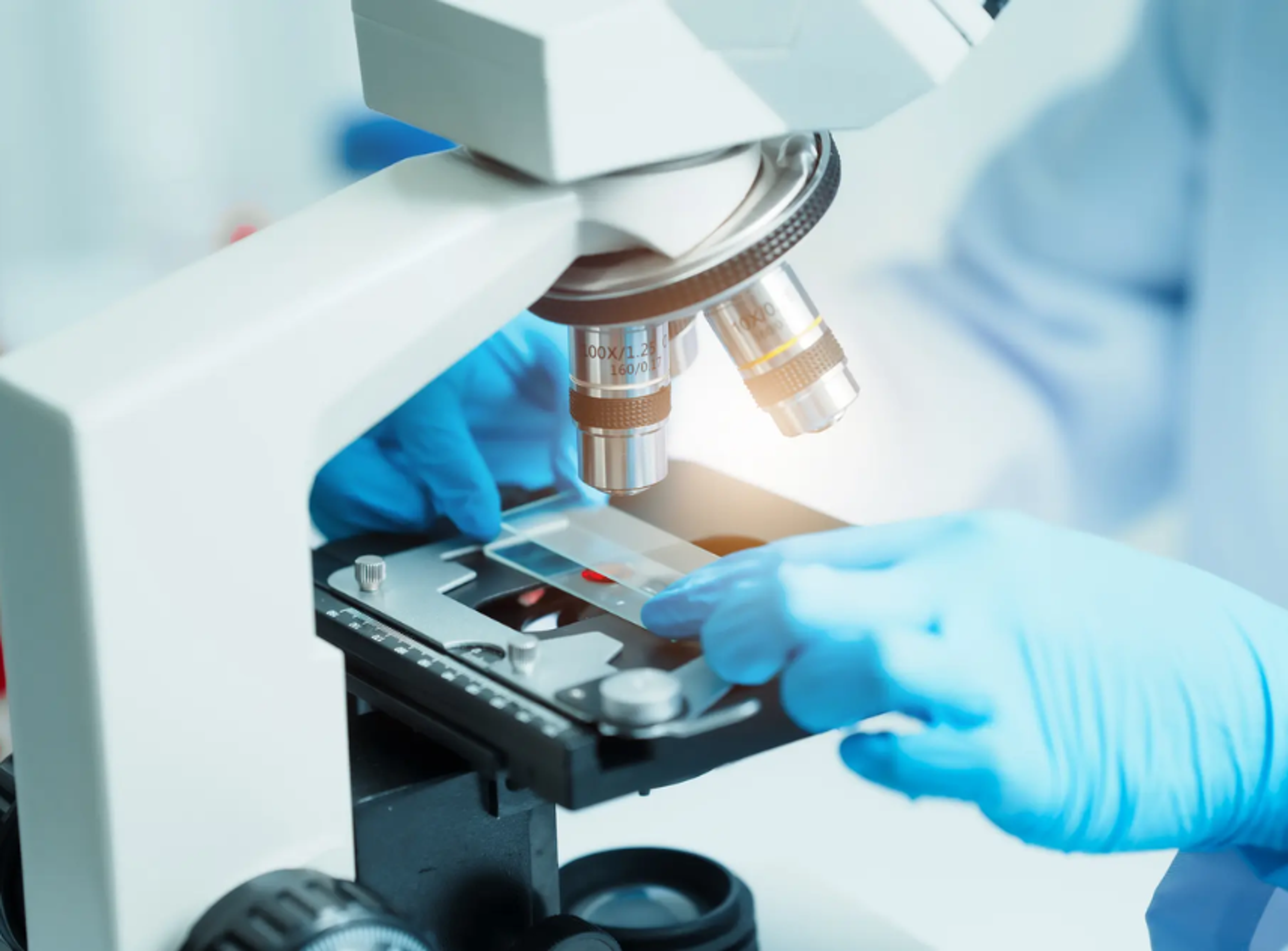
Choose the best microscopy equipment for your laboratory
Microscopy is a versatile and widely used technique with numerous applications across several fields of science and industry, playing a critical role in advancing research and solving real-world problems.
When purchasing microscopy equipment, you must consider several key factors to ensure you choose the right microscope for your lab's specific imaging needs.
In this 11th edition eBook, we present a “how-to-buy” guide that answers key microscopy questions and outlines the different microscopy approaches, including:
- Optical or light microscopy techniques such as widefield, confocal, and fluorescence microscopy
- Electron microscopy techniques such as scanning electron (SEM), transmission electron (TEM), reflection electron (REM), and scanning probe microscopy (SPM)
- Correlative and multimodal microscopy techniques, and more!
Enhance your imaging with the right reagents and tagging strategies
Selecting the right microscopy equipment is just the first step. Optimizing your imaging requires carefully choosing the appropriate reagents and labeling strategies. Fluorescent dyes, fluorescent proteins, and antibody-based labeling techniques are crucial tools that enable specific visualization of structures or dynamic processes with high precision. This guide also provides insights into various fluorescence tagging methods.
Download this eBook today and discover how to elevate your lab’s microscopy capabilities and make informed purchasing decisions.