Protein sequencing sheds light on protein misfolding diseases
With the help of next-gen protein sequencing tools, researchers have developed a new way to find, trap, and characterize altered protein shapes underlying multiple diseases
8 Aug 2024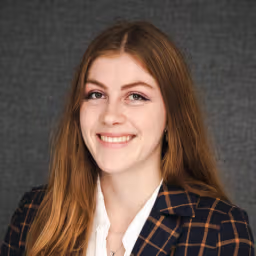
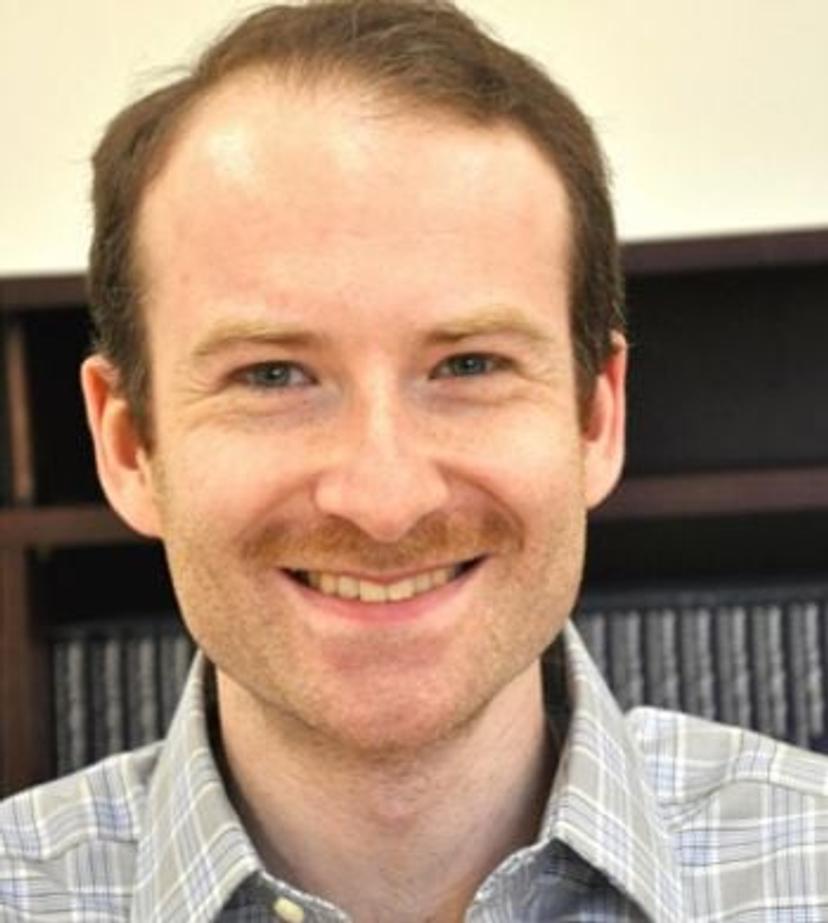
A team of researchers from Stony Brook University is taking a new approach to systematically discover protein conformations and directly link them to molecular, organismal, or evolutionary phenotypes. By combining protein barcoding and next-generation single-molecule sequencing on the Quantum-Si Platinum® instrument, the method offers a high-throughput means of mapping protein forms that cannot be crystallized and could help advance the treatment of neurodegenerative diseases and other disorders that result from protein misfolding.
We spoke with Eugene Serebryany, an Assistant Professor in the Department of Physiology and Biophysics and the Laufer Center for Physical and Quantitative Biology at Stony Brook University, to learn more.
Understanding the protein puzzle
Proteins – the chains of amino acids that orchestrate biological functions – exist in a wide range of distinct conformations (3D shapes), some of which play a pivotal role in driving disease pathologies. Protein misfolding, where a protein fails to adopt its correct 3D structure, has been implicated in multiple disorders, ranging from neurodegenerative conditions such as Alzheimer's, Parkinson’s, and Huntington’s disease to non-neurological diseases including cataracts, short-chain amyloidosis, and cancer.
A better understanding of the toxic effects and clearance of misfolded proteins may reveal novel strategies for the prevention and treatment of many diseases. However, elucidating disordered protein conformations has proven challenging with current experimental techniques, as misfolded proteins cannot be crystallized – a method commonly used to define the protein structures.
The approach developed by Serebryany and his team overcomes this limitation, enabling protein conformations generated in vitro or in vivo to be stabilized, purified, and subsequently sequenced to map their phenotypic effects. The essence of the method, called high-throughput disulfide scanning (HTDS), is to reduce the 3D problem of protein structure mapping to a 1D problem of protein sequencing.
High-throughput disulfide scanning of protein conformations
HTDS leverages the unique biochemical properties of disulfide bonds, bonds that form between cysteine residues and impose conformational constraints on protein shape. “We adapted disulfide mapping, which is a process where you put a pair of cysteine residues somewhere in the structure of a protein, and if the residues are close together, they will form a disulfide bond; and if they are far apart, they won’t,” explains Serebryany.
The likelihood of formation of disulfide bonds depends on the protein's folding dynamics, providing insights into the proximity and spatial organization of different parts of the protein structure. To reveal which disulfide bonds trap particular protein conformations, the researchers devised a deep sequencing method that could precisely and simultaneously locate both cysteine residues within each polypeptide across a library of double-cysteine protein variants.
“Using this technique, we can essentially unpack the 3D structural space of a protein into subsets of one-dimensional sequence space via disulfide cross-links and map whether or not a given pair of cysteine residue can covalently link to each other,” Serebryany says.
The approach has already been shown to enable disulfide scanning on an entire protein in a single experiment – a feat previously too impractical to attempt using conventional methods. Moreover, HTDS not only enables the assessment of the biophysical properties of protein conformations in vitro but it can also be used to evaluate the phenotypic consequences in vivo.
“We can transform these double cysteine scanning libraries into the host organism and determine, at the phenotypic level, which cells have greater fitness, which have lower fitness, which have different phenotypes that can be sorted, and which disulfide corresponds to those phenotypes,” Serebryany enthuses.
Improving HTDS with protein barcoding
In their initial study, Serebryany and his collaborators, then at Harvard University, relied on mass spectrometry for protein sequencing, which, while feasible, posed multiple challenges. “Mass spec is very good for point mutations, but with disulfide scanning, you need to make two mutations at a time in the same molecule, and you need to know the location in the sequence of both cysteines in the same polypeptide chain at the same time,” explains Serebryany.
The team devised a sophisticated approach that enabled chemical cleavage of proteins at specific cysteine sites, followed by mass spectrometric analysis of intervening peptides to ascertain from their termini the former cysteine locations. However, despite its utility, Serebryany notes several method constraints: it is limited to relatively short sequence distances between the introduced cysteines, and any cleavage in-between two introduced cysteines leads to a complete loss of structural information from that cysteine pair.
Exploring the vast conformational space of proteins via peptide barcoding required a new sequencing approach, and we turned to the Quantum-Si instrument to find it.
Eugene Serebryany
Stony Brook University
To surmount these limitations, the researchers are now looking to protein barcodes – short stretches of amino acids that can be used to uniquely label proteins. “These would allow the application of this technique to larger, more complex proteins and those with native cysteine residues,” says Serebryany. “We could create a library of peptide barcodes attached to one of the termini, and at the end of the experiment, simply cut off the barcode and sequence it.”
For protein barcoding to be practical for HTDS, barcodes need to be as short as possible and have similar amino acid composition and chemical properties to minimize the likelihood of differential effects on protein structure and folding. However, short barcodes with the same amino acid composition are difficult to sequence using mass spectrometry.
“Mass spec typically requires peptides longer than six residues for confident sequence determination,” notes Serebryany. Furthermore, interpreting and analyzing mass spectrometry data for libraries of peptides of the same mass or similar chemical properties is challenging due to overlapping mass spectra.
This is where Next-Generation Protein Sequencing™ (NGPS) on Quantum-Si’s Platinum® instrument comes in. “Single-molecule peptide sequencing is preferable to mass spec for analysis of libraries of short, chemically similar peptide barcodes,” says Serebryany. “This is what really piqued my interest in Quantum-Si, and we are now leveraging this technology for HTDS."
Taking protein barcoding to the next level
The Platinum® instrument is the first to enable protein barcode sequences to be read directly and identified with single-molecule resolution. It relies on unique kinetic signatures to differentiate and identify amino acid residues and post-translational modifications (PTMs), enabling the quantification of peptide sequences with high confidence. In doing so, the benchtop instrument introduces a convenient and user-friendly method for decoding peptide barcodes, opening avenues for multiplexed protein characterization, variant screening, and various other applications.
“There’s a lot of innovation here that can further advance our novel structural technique,” Serebryany enthuses. “Exploring the vast conformational space of proteins via peptide barcoding required a new sequencing approach, and we turned to the Quantum-Si instrument to find it.”
Future promise
Looking ahead, Serebryany sees great potential in the combination of HTDS and protein barcodes using Next-Generation Protein Sequencing™, particularly for drug discovery. “Peptide-barcoded HTDS could enable many types of biophysical measurements, such as thermodynamic stability, binding affinity to a target, or aggregation propensity, at much higher throughput than conventional methods,” he says. “Because HTDS stabilizes diverse conformations, it may prove valuable in applications such as vaccine design and drug screening.”
HTDS could also advance the treatment of neurodegenerative and other diseases that result from protein misfolding. “Stabilization of misfolded aggregation precursors via disulfide cross-linking could be used for designing protein vaccines,” Serebryany adds. “Another interesting application is the identification of disulfides that can trap non-native cytotoxic conformations. This could enable screening for drugs that select that conformation, offering the potential to create a new class of antibiotics that work by induced misfolding of target proteins.”
“There's a vast conformational space of proteins that remains to be explored due to a lack of high-throughput experimental methods. The combination of single-molecule sequencing and protein barcodes has the potential to dramatically expand this field of study,” Serebryany concludes.