Analytical measurements supporting cellular therapies
Explore the applications of cell counting through their context of use
17 Apr 2024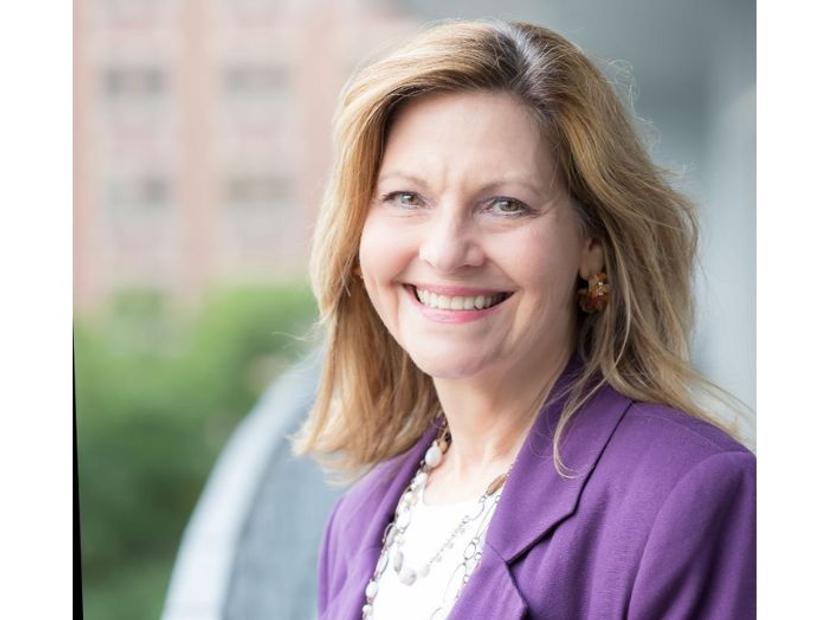
Dr. Virginia Litwin, Director, Scientific Affairs, Eurofins Clinical Trial Solutions
The decisions concerning how, when, and why laboratory measurements are made are dictated by their context of use (COU) (i.e. the intended use of the data and its associated regulatory requirements). Moreover, assay performance requirements are also mandated by the COU. This is true for complex assays such high-dimensional, spectral flow cytometry, as well as simple methods such as counting cells. In the development of cell therapies both types of assays are required. In this article, the influence of the COU on the cell counting measurements used for cell therapy manufacturing, development and clinical evaluation are discussed.
Introduction to cell and gene therapy
Cell therapy is one of the most exciting and promising new therapeutic modalities, encompassing both genetically engineered cells and normal cells derived from autologous or allogenic origin. Chimeric antigen receptor (CAR) T-cells are autologous, and more recently allogeneic, cells that have been genetically engineered to recognize cancer cells. The genetic engineering other cell types such as natural killer (NK) cells and monocytic cells are also under development. Most commonly the cells are manipulated ex vivo and infused into the patients however, novel in vitro gene modification approaches are under development. Non-engineered, or normal cell therapies include hematopoietic stem cells and allogeneic NK cells.
Cell therapy, CAR T-cells in particular, represent one of the most impactful scientific advances in cancer immunotherapy. These therapies are increasingly applied in clinical practice. CD19 CAR-T cells targeting B cell malignancies are resulting in impressive remission rates in patients1. The potential of CAR therapies in the treatment of solid tumors, autoimmune conditions and infectious diseases is currently being investigated.
Using flow cytometry, upwards of 30 measurements can now routinely be made simultaneously on thousands of single cells, relatively rapidly, and with exquisite sensitivity and specificity.
Cell therapy characterization
Living cells are a unique therapeutic modality requiring distinct analytical measurements compared to small molecule drugs and protein immunotherapies. Not surprisingly flow cytometry, the premier technology for single cell characterization, has become an indispensable analytical tool supporting all stages in the life cycle of a cellular therapy from manufacturing through to longitudinal evaluation of the infused cells in patients.
Using flow cytometry, upwards of 30 measurements can now routinely be made simultaneously on thousands of single cells, relatively rapidly, and with exquisite sensitivity and specificity2. The ability to achieve such high parameter measurements is due, in large part, to the advancement of spectral flow cytometry and the generation of novel fluorescent probes3. Briefly, and oversimplified, the field of single cell analysis has gained not only additional probes, but also instruments that can measure more of their signal with considerably lower background. To state this in an even more simplified way, more signal, less noise. Without doubt, these technological advances are helping to move the field of cell therapy forward by facilitating more detailed and reliable measurements, which require less clinical sample volume than the older flow cytometry technologies.
In the manufacturing of cell-based therapeutics, flow cytometric methods provide essential data for both the in-process optimization of the cell culture conditions and transduction efficiency of the genetically engineered cells, as well as for the final assessment of critical quality attributes (CQA) (viability, purity, identity, concentration, quality) required in product release testing. Typically, a variety of technologies are used for the CQA, but identity and purity are best evaluated using multiparametric flow cytometry4. It is important to monitor the phenotype of the cellular drug product as it progresses from manufacturing to delivery.
Living cells are a unique therapeutic modality requiring distinct analytical measurements compared to small molecule drugs and protein immunotherapies.
Post-infusion monitoring (cellular kinetics)
The fate of the cellular drug product post-infusion, determined both by the cellular drug’s phenotyping and enumeration of the number of cells in circulation, is correlated with clinical outcomes5. For CAR T cells, the expression of a large repertoire of T cell markers including differentiation, activation, exhaustion, and checkpoint molecules are routinely measured. When administering any type of immune therapy including CAR T cells, it is informative to evaluate the disposition of the patient’s immune cells in addition to the infused cells. Using high-dimensional, spectral flow cytometry, extensive phenotyping of both the resident and infused T cells can be conducted in the same sample, minimizing the sample volume requirements. This type of testing can be conducted in whole blood samples using a Lyse/Wash assay format or on isolated peripheral blood mononuclear cells (PBMC), as is well described in the literature6, 7.
While there is little controversy in the field regarding the optimal process for developing and validating complex, high-parameter, spectral flow immunophenotyping methods, when it comes to cell enumeration assays which generate an absolute count expressed, as the number of cells per unit volume, there is a surprising lack of understanding in the field.
It is well understood that extensive phenotyping must be performed in washed whole blood or isolated PBMC in order to maximally resolve the diverse populations. These populations, or cell subsets, are reported as the percentage of some parent population such as total lymphocytes or total T cells. The absolute counts of these populations can then be calculated using the absolute count of the parent population generated in a separate assay.
The best way to do this is to design a companion tube for cell counting to be used alongside of the phenotyping tube. The cell counting tube must contain counting beads if the flow cytometer is not equipped with volumetric counting capabilities. Most importantly the counting tube must use a Lyse/No Wash format given that absolute counts cannot accurately be generated in an assay in which the blood has been washed, as there is no way to assess the cell recovery after washing8, 9. The counting tube should contain only the monoclonal antibodies (mAb) required to specifically identify the parent population, but not the extensive panel of mAb used in the phenotyping tube. The counting tube should follow the same initial gating strategy as the phenotyping tube. Using this approach the parent population derived in the counting tube will be as close as possible to the parent population in the phenotyping tube and thus generate the most reliable results.
Other approaches for deriving the absolute count of the parent population are commonly used but they are not optimal. One commonly used approach is to derive the absolute count of lymphocytes from results generated on clinically approved hematology analyzer; while this will work, there are several drawbacks. One is that the hematology analyzer will likely require a different anticoagulant from that used for the flow cytometric method. This fact introduces variability between the results generated by the two methods as the anticoagulants may affect the cells in different ways; moreover, each tube may not be optimally mixed, one tube may contain small clots. Of greatest concern is that a hematology measures lymphocytes differently than the flow cytometric method.
The hematology analyzer is actually less specific and less sensitive than a flow cytometer. The hematology analyzer identifies lymphocytes solely based on light scatter properties; whereas an optimally designed flow cytometric method will identify the lymphocytes based on light scatter and specific mAbs such as CD45 (lymphocytes are bright for CD45) and CD14 (lymphocytes are negative for CD14).
In addition, light scattering technology is more sensitive on a flow cytometer than a hematology analyzer. Lastly, the flow cytometric method will only include single cells events (i.e. excluding doublets) in the lymphocyte count.
A second commonly used approach is to take the absolute count of lymphocytes or T cells generated from an IVD/CE marked clinical diagnostic flow cytometric assay for the major lymphocyte populations (T cells, B cells, NK cells). While this approach is preferred to using a hematology analyzer, it still presents some limitations, which in the end, compromise the final results.
Although the IVD/CE marked methods are considered the gold standard, they actually rely on older flow cytometry technology and have not been updated to take advantage of many technological advances. Thus, the IVD/CE marked methods actually generate less specific data than the custom approach described above. They rely only on CD45 and light scatter for lymphocyte identification. The addition of CD14 has been shown to greatly improve the specificity of the lymphocyte gate. Furthermore, they do not include doublet discrimination.
When administering any type of immune therapy including CAR T cells, it is informative to evaluate the disposition of the patient’s immune cells in addition to the infused cells.
Summary
In order to best support the development of cell-based therapies the testing laboratories should strive to generate the most reliable data. There are not clear directives from regulatory agencies regarding testing to support drug development or for many clinical laboratory testing scenarios. It is thus up to the laboratorians to develop the most robust, state-of-the art methods for each particular COU10.
This article was written by Dr. Virginia Litwin, Director, Scientific Affairs, Eurofins Clinical Trial Solutions.
Learn more about Eurofins' integrated flow cytometry and the creation of its new flow cytometry centers of excellence.
References
Lee W.H., Graham C.E., Wiggin H.R., Nolan H.K., Graham K.J., Korell F., Leick M.B., Barselau A.L., Emmanuel‐Alejandro E., Trailor M.A., Gildea J.M. Optimization of a flow cytometry test for routine monitoring of B cell maturation antigen targeted CAR in peripheral blood. Cytometry Part B: Clinical Cytometry. 2024 Feb 28.
Sommer U., Eck S., Marszalek L., Stewart J.J., Bradford J., McCloskey T.W., Green C., Vitaliti A., Oldaker T., Litwin V. High‐sensitivity flow cytometric assays: considerations for design control and analytical validation for identification of rare events. Cytometry Part B: Clinical Cytometry. 2021 Jan;100(1):42-51.
Mitra-Kaushik S., Mehta-Damani A., Stewart J.J., Green C., Litwin V., Gonneau C. The evolution of single-cell analysis and utility in drug development. The AAPS Journal. 2021 Aug 13;23(5):98.
Sarikonda G., Mathieu M., Natalia M., Pahuja A., Xue Q., Pierog P.L., Trampont P.C., Decman V., Reynolds S., Hanafi L.A., Sun Y.S. Best practices for the development, analytical validation and clinical implementation of flow cytometric methods for chimeric antigen receptor T cell analyses. Cytometry Part B: Clinical Cytometry. 2021 Jan;100(1):79-91.
Mueller K.T., Waldron E., Grupp S.A., Levine J.E., Laetsch T.W., Pulsipher M.A., Boyer M.W., August K.J., Hamilton J., Awasthi R., Stein A.M. Clinical pharmacology of tisagenlecleucel in B-cell acute lymphoblastic leukemia. Clinical cancer research. 2018 Dec 15;24(24):6175-84.
Ferrer‐Font L., Pellefigues C., Mayer J.U., Small S.J., Jaimes M.C., Price K.M. Panel design and optimization for high‐dimensional immunophenotyping assays using spectral flow cytometry. Current protocols in cytometry. 2020 Mar;92(1):e70.
CLSI (Ed.). (2021). Validation of assays performed by flow cytometry, 1st ed. CLSI document H62.Wayne, PA: Clinical Laboratory Standards Institute.
O'Hara D.M., Xu Y., Liang Z., Reddy M.P., Wu D.Y., Litwin V. Recommendations for the validation of flow cytometric testing during drug development: II assays. Journal of immunological methods. 2011 Jan 5;363(2):120-34.
Wood B., Jevremovic D., Béné M.C., Yan M., Jacobs P., Litwin V., ICSH/ICCS working group. Validation of cell‐based fluorescence assays: practice guidelines from the ICSH and ICCS–part V–assay performance criteria. Cytometry Part B: Clinical Cytometry. 2013 Sep;84(5):315-23.
Mathews J., Amaravadi L., Eck S., Stevenson L., Wang Y.M., Devanarayan V, Allinson J., Lundsten K., Gunsior M., Ni Y.G., Pepin M.O. Best practices for the development and fit-for-purpose validation of biomarker methods: a conference report. AAPS Open. 2022 Feb 1;8(1):2.