Nuclear Fusion: The Pursuit of Limitless Energy Inspired by the Sun
Dr. Alexandru Boboc’s fusion experiments stand the test of demanding experimental conditions for a pressing future goal
4 May 2017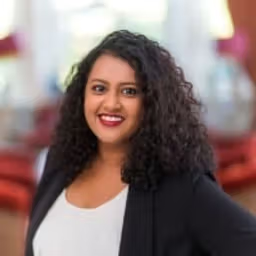
It’s the 1950s. A few scientists ask a bold question: what if we could build a sun on earth? It would solve our biggest problem – limited supply of energy. The bright and powerful sun has virtually limitless energy.
Unlike our worries of exhausting fossil fuels on earth, or our incapacity to harness solar power on a cloudy day, the sun is self-sustaining and on the job 24/7.
Over 60 years later, physicists believe recreating the sun inside a lab is just around the corner. SelectScience® caught up with one such scientist, Dr. Alexandru Boboc, from the Culham Center for Fusion Energy (CCFE), U.K., about his mission to benefit the future generations by exploiting the sun’s trade secret – nuclear fusion.
How does the sun do it?
To seek inspiration from the sun, Dr. Boboc and his colleagues first consider how the sun works. Two small atoms combine to form one large atom nucleus, releasing energy in what is the basic equation of nuclear fusion. As we already know, an atom consists of a positively-charged nucleus surrounded by negatively-charged electrons. How, then, do the atoms fuse? “For fusion of two atoms’ nuclei, very high energies and temperatures are required. In these special conditions, matter becomes, what is called, the plasma state,” explains Dr. Boboc.
As it turns out, the immense heat and enormous gravity in the sun’s core generates plasma, the fourth state of matter after solid, liquid and gas. In a plasma state, the electrons are stripped off their atomic nuclei and float around. The nuclei, now separated from their electrons and all positively charged, repel one another, resisting fusion. The sun, again, has a solution to this: its insides are compressed under a lot of pressure driven by its own massive mass. This pressure is so high, and the temperatures so hot, that even like-charged nuclei squeeze together and fuse, releasing energy in the process.
Man's version of sun
I. Plasma
To replicate the sun’s nuclear fusion, scientists need to first create plasma. As a part of the Joint European Torus (JET) at the CCFE, Dr. Boboc, along with the scientists working on the JET fusion experiment, employ a doughnut-shaped vacuum chamber surrounded by magnetic coils called the tokamak where the plasma resides. In the tokamak, the plasma is generated using electric currents and magnetic fields, whereas the sun uses its own high temperature and pressure for the same. “The plasma temperatures are tens of millions of degrees Celsius – many times hotter than the sun’s core,” says Dr. Boboc. “Actually, in 1969, a team from CCFE was the first to measure a plasma temperature of 11,000,000°C.”
The periphery of the most modern tokamak is lined with superconducting magnetic coils which operate at yet another temperature extreme: -269oC. This experimental setup hosts the largest temperature gradient in the universe within the range of one meter. “This is not science fiction – we’ve been doing it for more than 50 years!” Dr. Boboc quips.
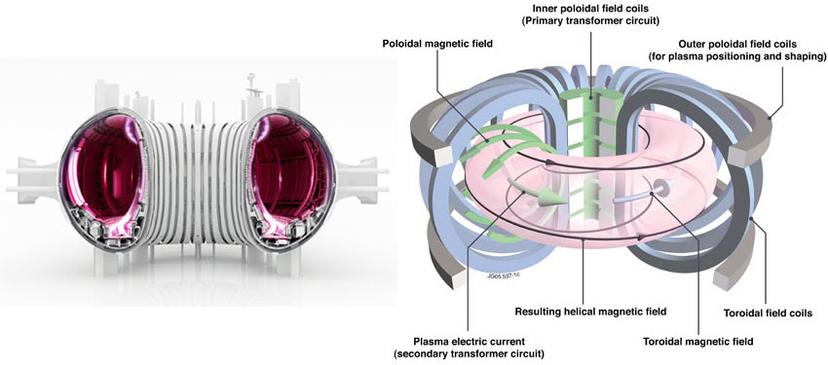
(Left) An artistic representation of the doughnut-shaped magnetic confinement reactor or tokamak. (Right) A schematic of the electric and magnetic fields that create the plasma. [Images courtesy of CCFE.]
II. Nuclear fusion
For nuclear fusion in the tokamak, the JET scientists fuse two hydrogen isotopes – deuterium and tritium. This choice of starting materials is very sustainable because these isotopes are readily available: deuterium can be easily found in sea water, and tritium – although rare as itself – can be made from lithium, the element that powers your laptops and cellphones.
With deuterium and tritium inside the tokamak, the electric current creates a plasma state of matter, and the magnetic fields compress the plasma such that its temperatures become high enough to finally achieve nuclear fusion.
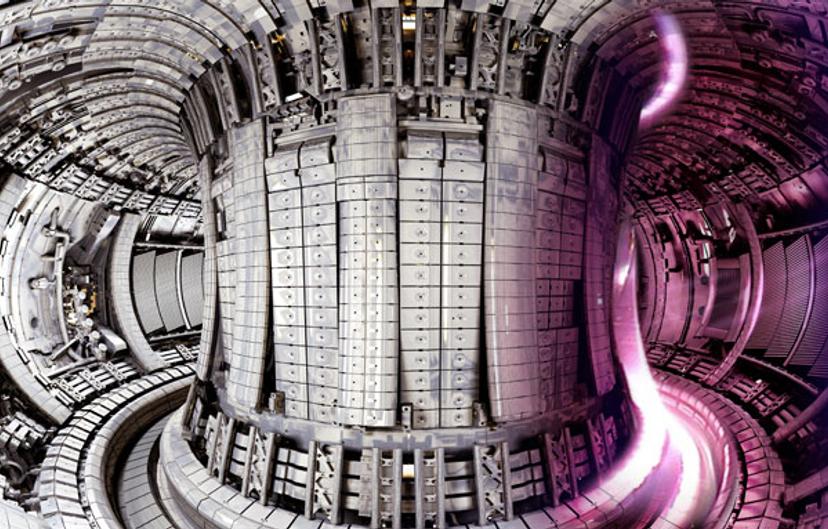
An artist’s rendition of the insides of a tokamak. Using deuterium and tritium as starting materials, the electric current creates a plasma state (shown here in pink). The magnetic coils lining the walls of the tokamak elevate the plasma to temperatures of millions of degrees Celsius, finally enabling nuclear fusion. [Image courtesy of CCFE.]
Dr. Boboc adds, “The European JET experiment, located at CCFE, is currently the largest fusion experiment in the world, and the only one, currently, that can do deuterium-tritium reactions. This particular reaction is the most efficient one for future energy production.”
III. Controlled conditions
Nuclear fusion needs close monitoring. It is especially important to examine the state of high-temperature plasma inside the tokamak so as to ascertain the fusion itself. But how would anyone ever get close to something that’s many times hotter than the sun?
“The working conditions inside a fusion reactor pose the most extreme engineering challenges that humankind ever had to cope with,” reasons Dr. Boboc. “Due to extreme conditions, there is no way to insert any device without risking damage to it or the plasma. So, most of the diagnostic systems are optical systems.”
Although humans or devices can’t get anywhere near the plasma, lasers can.
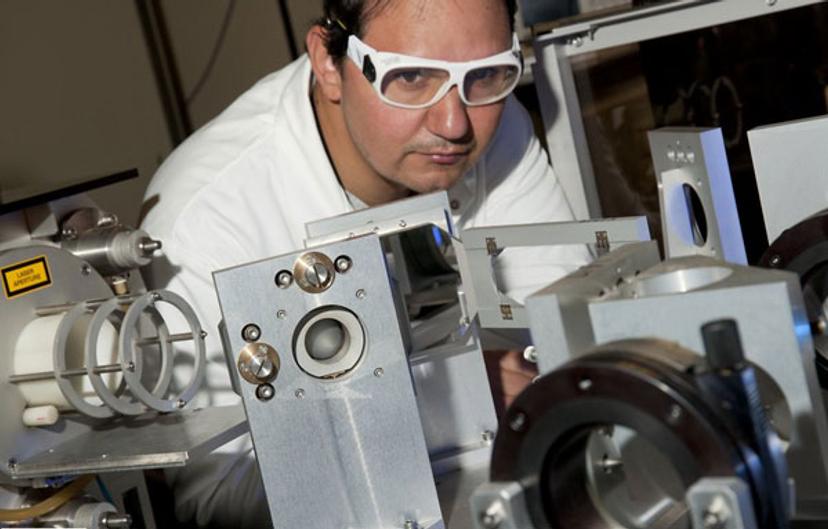
Lasers can go where no man or machine can. Dr. Alexandru Boboc aligns a far infrared optical laser used to study plasma properties. [Image courtesy of CCFE.]
To study plasma properties such as plasma density, Dr. Boboc projects two types of infra-red laser beams into the plasma. As soon as the beam hits the plasma, its optical property changes. The change in the laser’s properties or its speed is a direct reflection of the plasma density and magnetic field structure. Such a technique is called polarimetry.
To regulate the laser beam’s properties before it strikes the plasma, the team relies on wire grid polarizers from Specac. Made of parallel metallic wires arranged at specified widths, the wire grid polarizers are placed along the laser beams. “Each (laser) beam line has more than one Specac polarizer as it is critical to ensure polarization of the beams at various locations,” says Dr. Boboc. “All the wire grid polarizers on the JET diagnostics were provided by Specac – on standard and custom mounts. Some of them are here from 1987. That makes them 30 years old!”
For experiments with such extreme temperatures and results that challenge the status quo every day, Dr. Boboc and his team place a high premium on top-quality instruments and robust performance. “Our experience with Specac has proven to be excellent over all these years. Their engineers always supported us for any requirements we had, such as installing wire grids on complicated custom mounts,” Dr. Boboc acknowledges. The Specac polarizers, in Dr. Boboc’s words, have remained stable even while sitting very close to the scorching plasma – just outside the vacuum chamber – in a high radiation environment.
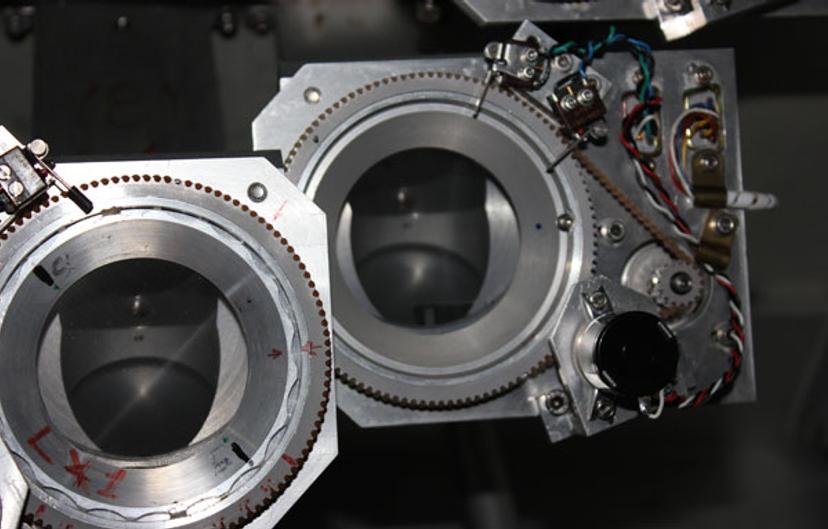
Specac’s wire grid polarizers participate in the fusion experiment at CCFE. The 10-micron wire grid polarizers from Specac, with tungsten wire grids, polarize a Deuterated Cyanide Gas Laser that Dr. Boboc uses to examine plasma density and magnetic field structure. (Left) A 30-year-old Specac wire grid from 1987, (Right) A recently purchased Specac grid on a custom mount. [Image courtesy of CCFE.]
IV. What lies ahead
- The return on (energy) investment
As with any transaction, a successful outcome depends on the return on investment. One of the biggest challenges with nuclear fusion is that it takes tremendous input energy to operate and maintain the electric currents and the magnets, but hasn’t yet yielded an output energy that is sustainable. Simply put, we’ve invested vast resources into running the machine and haven’t reaped energy from it. Well, at least not yet.
Recently, a consortium of 35 countries have invested in what is the most ambitious energy project ever — the ITER. Being built in southern France, the ITER aims to finally yield feasibility from a nuclear fusion reactor on a large-scale operation. Over 200 tokamaks from around the world, including the one in CCFE that Dr. Boboc works with, have acted as building blocks for the grand ITER conception. Dr. Boboc contributes as an expert in plasma diagnostics with the ITER.
- An automated reactor
In the near future, researchers hope to establish a type of ‘key operation’, like that used in our cars, inside a fusion reactor. “Currently, I’m working on a nearly total automation to create a ‘key-operated’ system using standard off-the-shelf components,” explains Dr. Boboc. In 2014, Dr. Boboc was the first to implement a real-time infrared polarimeter that has been used unattended for the safety and control of a nuclear fusion plant.
“We do now know how to make fusion, we do know how to control it. What remains is to solve some stringent engineering issues on materials, and complete ITER on time and within budget as the next major step,” reckons Dr. Boboc.
“I personally strongly believe that the fusion community has reached the point of no return, that we can deliver the first commercial plant prototype before 2050 if there will be enough political and financial support,” Dr. Boboc concludes, with a familiar kind of cautious optimism and strong passion that has long bolstered nuclear science advancement since the 1950s.