Nitrogen carrier gas: A viable alternative to helium
Guest editorial: Frank Dorman, Senior Global Market Manager, Waters Corporation
27 Jan 2023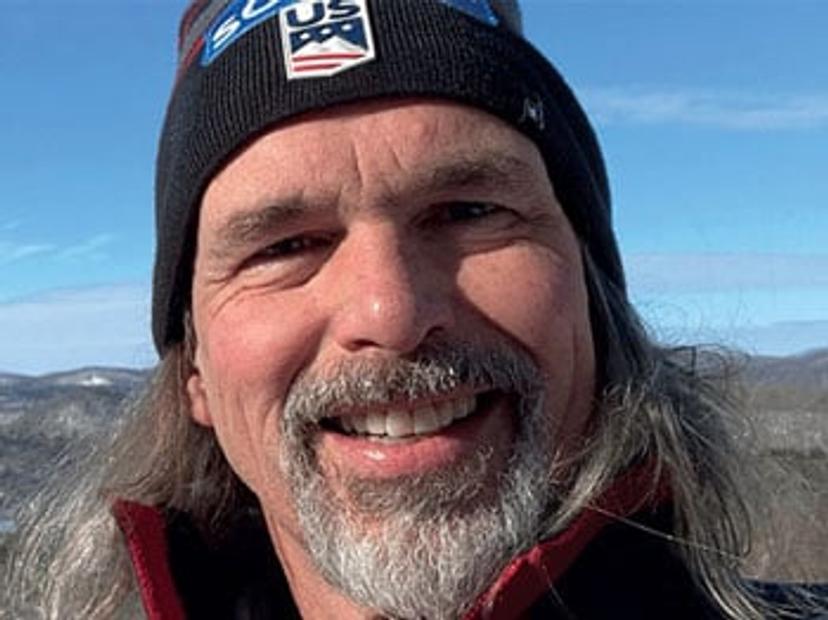
“Those who do not learn from history are doomed to repeat it”. How many have heard that saying? Unfortunately, history is not always correct in the field of gas chromatography, especially if you only look back a few years to a time when instruments were not reflective of modern instrumentation. This is the case with common choice of carrier gasses for GC and GC-MS separations and it has resulted in an essentially singular use of helium in all but a very few analytical separations. Additionally, most GC-MS systems have evolved to the point of where they are effectively optimized for use with helium carrier gas. This affects things like the vacuum pumps that are commonly used for the benchtop systems as well as some of the onboard electronics and filtering devices that are constructed with the assumption that the end user is using helium for their mobile phase. Switching from that has been much less common over the past couple decades since helium has, mostly, been available even though the cost has steadily increased relative to other common laboratory gasses.
Over the past few decades, occasional helium shortages have caused concerns to laboratory managers. Typically, with each shortage the price of helium has increased significantly and remained there even after the shortage has subsided. As a result, helium prices are currently 10-fold higher than nitrogen, for example. Even hydrogen is 2-5 times less expensive than helium for comparable GC-compatible carrier gases. Actual prices vary depending on location, contracts, and usage, but nitrogen is always considerably less expensive than helium. I’m sure that many readers are now thinking, however, that nitrogen is not compatible with the needs of gas chromatography and they may even use “history” to back this opinion up, but the key to the initial statement in this article is learning from history and merely recalling what has been promoted by some is not learning. In short, nitrogen is a very useful gas as an alternative to helium as a GC carrier and it has a number of very valuable properties that could arguably make it preferred to helium and certainly to other options that a chromatographer may choose.
First, why discuss this subject now? I’m sure readers are aware of the current growing issue with helium. There is a global shortage of it and as a result costs have risen dramatically in many areas of the globe.[1] Maybe more importantly than cost, when helium is in short supply laboratories shut down instruments and stop certain analyses. Helium is a byproduct of gas wells. Purifying helium requires specialized infrastructure and not all gas wells are equipped for its recovery. Due to challenges in the global supply of helium as a result of difficulties in the hydrocarbon gas manufacturer arena, helium supply has been inconsistent over the last several years. Maybe even more importantly, there are a number of other uses for helium that impact availability to laboratories. Party balloons , medical diagnostics instruments and superconducting magnets consume a considerable amount of the helium supply and bleed off gas supply to chromatographic use which is a source of the problem.
So, assuming that the reader is a gas chromatographer and is interested in breaking the reliance on helium for their separations needs, where might one turn to for information for alternatives? Considering that most commercial manufacturers of GC instrumentation have optimized their systems for helium use, the chromatographer might not get a complete story from them on the use of other gasses. Additionally, if the reader gets information for materials (textbooks, literature, etc.) that were developed a number of years ago when GC separations were commonly performed using constant pressure carrier gas control, they may make assumptions that are no longer valid with more modern instrumentation. Here are where the van Deemter curves enter the picture.
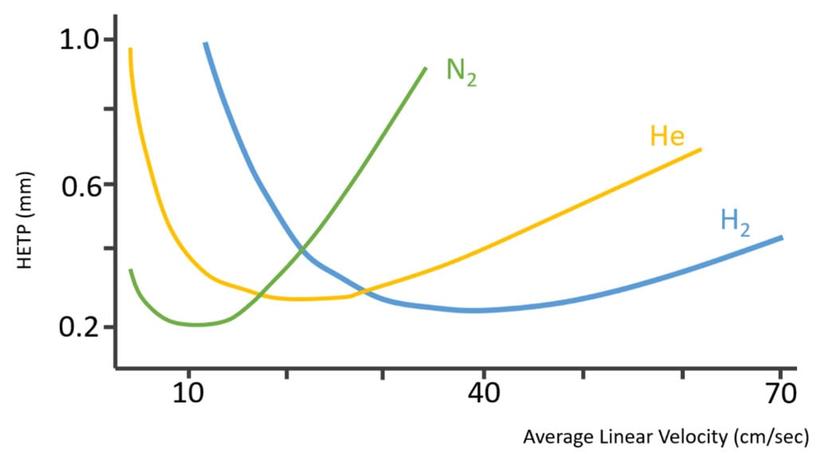
Most readers will be very familiar with this figure. It is used to set the optimum linear velocity for helium and other gasses and for setting the initial column flow rate when using pressure control. As a result of a narrower range of optimum linear velocity (flow rate), nitrogen was often not preferred when using constant pressure when in temperature programmed mode. As the oven temperature increases, the carrier gas viscosity also increases, and this causes the linear velocity to slow down, again when operating in constant pressure mode. If using a wide range of temperatures, nitrogen can be less efficient more quickly as a result of this narrow range of optimal velocity. Nitrogen then often was said to be “slow”, as compared to helium or hydrogen, as its optimum linear velocity occurs at a lower value than the other gasses, but there are many factors that are not considered in this relatively simple explanation of the relationship between linear velocity and efficiency (height equivalent to a theoretical plate) that is plotted in a van Deemter curve. For example, it is rarely pointed out that nitrogen actually is capable of higher efficiency at its optimum linear velocity than the other gasses, potentially allowing a chromatographer to achieve a bit higher resolution between closely eluting peaks. Possibly more important, however, is that this type of plot does not consider the effect of changing column dimensions as a user changes from one carrier gas to another and what effect that has on the resulting separation. Lastly, the van Deemter plot does not address the issue of chemical reactivity, which is a big reason hydrogen raises concerns as a choice for a carrier gas.
While hydrogen’s flammability is a point of concern, with proper safety controls, this can be managed. The larger issue is that hydrogen is a reducing gas and it is not chemically inert. Many will be familiar with hydrogenation reactions which are often covered in first-semester organic chemistry classes.[2] Many functionalized molecules can undergo hydrogenation, and the higher temperatures and potential catalytic surfaces encountered, especially in GC-MS, can cause spectral differences in their compounds when comparing helium-based separations to those performed using hydrogen. Hydrogen also decreases sensitivity on most GC-MS systems, likely due to less efficient pumping with the types of pumps used on modern commercial systems. As a result, it is arguably not the best choice unless the user is left with no other options.
So, what can be done to maintain sensitivity, separation quality and overcome supply issues? The answer is - nitrogen. Additionally, nitrogen is a renewable gas, so it is more friendly in terms of how it is obtained, separated, purified, and delivered. It may even be able to be supplied, with necessary filtration, using laboratory gas generators which could possibly eliminate the need for high-pressure cylinders altogether.
For GC separations using non-MS detectors, nitrogen is a completely viable alternative, especially if column dimensions are scaled (discussed below). Unfortunately, due to how most commercial GC-MS instrumentation has been optimized around the use of helium, nitrogen is not always a viable alternative. On the smaller bench-top electron ionization (EI) systems, nitrogen often produces a significant background which decreases overall signal-to-noise, and hence sensitivity suffers. Additionally, the vacuum pumps are not really designed to operate with nitrogen carrier gas and in some cases the use of nitrogen may void a manufacturer’s warranty with regard to the pumping systems. On the other hand, for GC-MS analyses with atmospheric pressure ionization (API), nitrogen is an excellent choice of a carrier gas and it does not result in a negative impact to sensitivity. If the chromatographic column is correctly scaled it is also possible to get the same quality separation as quickly as one would using helium. Since modern instruments regulate flow rate, the relatively narrow range of highest efficiency observed in the nitrogen van Deemter curve is also no longer a concern.
Taking a closer look at one commercial option for atmospheric pressure ionization GC-MS, the Waters APGC ion source operates using only nitrogen. It is designed for that purpose and as such it has no limitations with regards to the use of nitrogen as a GC carrier gas. The API source chosen for this study uses a nitrogen plasma for initial ionization of what elutes from the GC. In some cases, it then performs a direct charge transfer from the nitrogen plasma to the compounds eluting from the GC column, and in other cases it is used to first ionize a reagent gas (think APCI on an LC-MS system) such as water vapor, which then in turn ionizes the compounds of interest. These two ionization processes are referred to as “dry” and “wet” source, respectively. Control of the ionization process is affected by what is added to the ion source as a reagent, or not, and source conditions, but this can be thought of as a “mixed mode” ion source that can perform either charge transfer (as in done in electron ionization (EI) sources, albeit at a reduced energy) or adduct ionization (as would be done in APCI). This enables the user to have more flexibility that is had with just conventional EI as well as increased sensitivity due the different ionization process and the manner in which ions are transported to the mass analyzer.
Separations with nitrogen as compared to those performed with helium are comparable as long as the physical dimensions of the GC column are scaled. Using the same column dimensions, changing from helium to nitrogen results in either a longer analysis time, or reduced chromatographic efficiency, but if the GC column dimensions are scaled down, then the separation with nitrogen can achieve the same separation time and resolution as was obtained using helium. The analyst can really do either of these, but there is a benefit to scaling the separation as then the resulting nitrogen carrier chromatogram is exactly the same as it was under helium carrier. Scaling the GC column dimensions down does reduce the loading capacity, but since the APGC ion source is so much more sensitive than an EI source, users typically adjust their injection volumes or injection conditions so that they are transferring a lower amount to the GC column while still easily meeting their reporting requirements, so this leads to a benefit as well – system robustness.
Figure 2 is an example chromatogram obtained from a helium carrier separation as compared to a nitrogen carrier separation using an APGC system for a series of later-eluting permethrins. As observed in the figure, with proper scaling of the GC column dimensions and adjustment of linear velocity, essentially equivalent separations can be produced using either gas. In the helium example, a 30M X 0.25 mm i.d. X 0.25 um df 5-type column was utilized. For the nitrogen example, a 20M X 0.15 mm i.d. X 0.15 um df column was used with the equivalent stationary phase. The minor difference in retention times is due to minor differences in actual versus nominal column length and id and could be adjusted to be exactly the same with a small adjustment in flow rate. This figure demonstrates, however, the simplicity of just changing the carrier gas and scaling the column to yield the separation shown.

Figure 3 demonstrates the same effect but for early eluting compounds. Again, the columns used for this example are the same as in Figure 2 and the figure represents what is obtained merely by scaling the column dimensions and adjusting the linear velocity to maintain efficient operation for the two different carrier gasses.
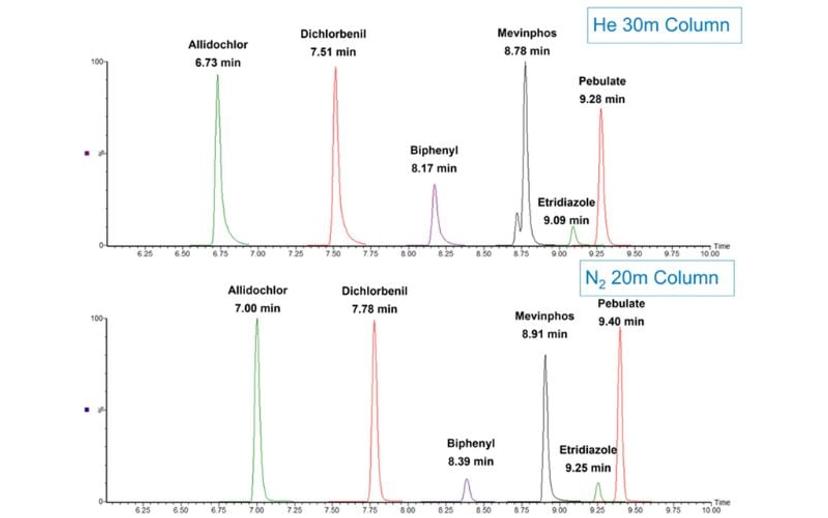
Interestingly, the peak symmetry appears to be improved for the nitrogen example for the early eluting components, which may have to do with the reduced linear velocity for the nitrogen separation allowing for a more effective sample focusing as compared to helium. Again, retention times could be matched exactly, if a further adjustment was made to account for the difference in actual versus nominal column dimensions, but this figure demonstrates the ease of switching from helium to nitrogen.
In summary, the use of helium is an increasing concern due to the difficulties in supply and the increasing cost. While hydrogen may provide an option for some users, it is problematic especially since it is not an inert gas. Nitrogen is both inert and also capable of equivalent separation performance as compared to helium and it is completely compatible with API ionization. While the benefits of API ionization are beyond the scope of this article it does provide benefits relative to EI-based systems and when coupled with the helium shortage concerns should be an important technology for the next generation of GC-MS systems.
References:
1. “The world is running out of helium. Here’s why doctors are worried”, NBC News, Caroline Hopkins, Oct 22, 2022.
2. “Organic Chemistry”, John McMurry, Wadsworth, Inc., Belmont, Ca, USA, ISBN 0-534-01204-3